Modeling for Device Design and Personalized Treatment Planning
Problem Description
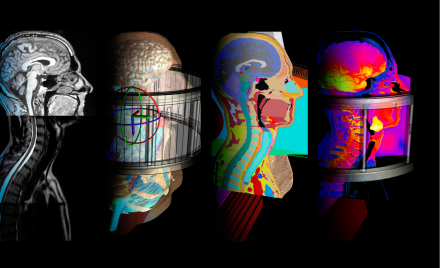
Various views of a simulation of a tumor being exposed to electro-thermal therapy. From left to right: Medical image of the problem; CAD model of the simulation set-up; cross section of the discretized model, simulation results with Sim4Life.
Hyperthermia cancer therapy (hyperthermic oncology) is used in combination with radio- and/or chemotherapy to treat a wide range of cancers. It employs electromagnetic energy to mildly heat a tumor, frequently resulting in dramatic improvement of initial response and survival rates. For deep seated tumors, phased radiofrequency (RF) arrays are typically used to target the energy to a tumor while avoiding overexposure of sensitive healthy tissues. Due to the strongly inhomogeneous nature of the human body and the impact of physiological factors such as blood perfusion and thermoregulation this is a demanding task. Simulations are required i) to personalize treatments reflecting tumor shape and location as well as the individual anatomy, and ii) to develop and investigate novel applicators capable of achieving controlled energy deposition in challenging locations such as the head and neck area.
RF and MW (microwave ) ablation use interstitial (inserted in the tissue, e.g., by catheter) applicators to locally heat tissues to high temperatures resulting in direct cell killing to treat diseases, such as cardiac arrhythmia or cancer. Alternative sources of ablation energy include ultrasound. Modeling is used to determine the impact of nearby vasculature on the achievable ablation zone, to optimize catheter placement, and to design novel applicators.
The required modeling functionality includes personalized model generation, electromagnetic and thermal simulation considering in vivo physiological factors, optimization of steering parameters, and outcome-related effect assessment.
Methodology
1. Patient Model

Generation of a personalized model from medical image data, with the iSEG module of Sim4Life.
Sim4Life supports the generation of personalized models from medical image data, e.g., for treatment planning. A wide range of image data, such as MRI and CT images, can be imported and jointly visualized with the simulation model and results (IMG module). The integrated image processing and segmentation module iSEG facilitates the rapid generation of anatomical models by offering a wide range of segmentation algorithms, ranging from highly interactive to automatic, which can be flexibly combined and are complemented by preprocessing routines to improve the image quality and measurement/analysis routines. The segmented images are then converted into surface-based body models suitable for simulation purposes using Sim4Life functionality that guarantees high quality, self-intersection-free, topologically compatible mesh creation.
When it is not necessary to employ personalized models, e.g., for mechanism investigations, applicator development, or when it is considered sufficient to personalize tumor location and shape but not anatomy, the highly detailed Virtual Population (ViP) 3.0 models, which have also been generated with, iSEG can be used instead.
2. 2. EM-Induced Tissue Heating

Simulation of electromagnetic exposure of the cancerous tissue, in Sim4Life.
Initially, the electromagnetic energy deposition is determined. In Sim4Life, the simultaneous setup of multi-antenna simulations is facilitated by the multi-port simulation feature, while flexible coherent and incoherent field combining is part of the analysis functionality. Depending on the frequency and the material properties, the full-wave P-EM-FDTD or the quasistatic P-EM-QS solver is best suited for determining the EM fields. In hyperthermic oncology, it is typically the former, while ablation modeling typically benefits from the latter. All of the EM solvers included in Sim4Life are optimized for simulation of setups comprising complex anatomical models, such as those of the ViP3.0. Sim4Life also facilitates the use of the integrated tissue properties database for assignment of dielectric properties.
Applicators and antennas can be designed with the Sim4Life modeler CAD functionality.
In vivo EM induced heating, both transient and steady state, is simulated with the P-THERMAL module. It is based on the Pennes Bioheat equation and accounts for metabolic and EM heat sources, heat diffusion, and heat transfer by tissue perfusion, with functionality to consider local thermoregulation by vasodilation as well as body core temperature increase over time. Convective surface cooling by external and internal air or a heated/cooled water-bolus in contact with the skin as well as the influence of large vessels in the treatment region can be included. Again, the Sim4Life tissue properties database includes thermal and perfusion parameters for a wide range of tissues.
3. Treatment Optimization

Model of an exposure set-up with a body model including the cancer, and a phased array applicator for electro-magnetic treatment. The treatment settings are optimized with Sim4Life.
For phased array applicators, such as in deep hyperthermia treatments or ablation treatment with multiple catheters, the phases and amplitudes of the individual antennas are optimized to achieve optimal tumor coverage while avoiding exposure of sensitive tissues. SIm4Life offers automatic and rapid treatment parameter optimization and allows the definition of multiple treatment regions as well as weighting to reflect treatment priority and tissue sensitivity. Alternatively, manual optimization is possible by means of the field combiner tool, e.g., while superposing the computed energy deposition distribution for a certain set of steering parameters over the medical image data of the patient.
Modeling can also be used to investigate and optimize the impact of other treatment parameters. For example, the impact of water-bolus temperature for surface cooling or heating is often optimized for individual treatment.
SEMCAD X / Sim4Life thermal therapy planning tools are in use in a series of leading clinical centers world-wide.
4. Effect Assessment

Assessment of the thermal treatment dose in treatment optimization with Sim4Life.
Induced in vivo effects are either predicted directly or assessed through thermal dose. A variety of tissue damage models is available in Sim4Life (T-CEM43): The Arrhenius model directly assesses tissue damage in coagulation and necrosis zones in ablation therapies. In hyperthermic oncology, the use of the CEM43 thermal dose concept is common. The CEM43 thermal dose expresses the thermal history of a tissue location in terms of minutes of heating at 43oC that would result in an equivalent thermal effect. It offers the advantage of not depending on tissue specific material parameters, and that CEM43 thresholds for thermal damage have been experimentally determined for a wide range of tissues and biological effects. Correlations between CEM43 and treatment outcome have been established clinically.
5. Device Design

Sim4Life used in design optimization of an hyperthermic applicator.
Sim4Life and SEMCAD X have been used to investigate various aspects of hyperthermic oncology applicators and RF/MW-ablation catheters, such as:
- optimal 3D arrangement and placement of antennas to achieve ideal focusing and steering potential in phased array deep hyperthermia systems
- exploration of arrangements featuring multiple ablation catheters and electrodes for superior focus steering and lesion shaping
- design of antennas with high efficiency and low loading dependency
- design of applicator elements that permit online determination of effectively applied phase and amplitude and cross-talk between antennas, allowing high treatment administration quality and feed-back control
- development of quality assurance phantoms and measurement setups
A range of novel applicators have been built and introduced into the clinic, including novel head & neck treatment applicators with superior focus control, MRI compatible applicators, waveguide-based superficial hyperthermia treatment systems, modular treatment applicator concepts for maximal usage flexibility, and applicator elements with integrated on-line monitoring and feed-back control.
6. Validation


Simulation of thermal therapies with Sim4Life has been accompanied by a range of verification and validation efforts. Experimental validation setup (top) and simulation (bottom).
Simulation of thermal therapies with Sim4Life has been accompanied by a range of verification and validation efforts. The electromagnetic and thermal solvers have been systematically verified for correct implementation of the physical and numerical phenomena of the underlying mathematical model. Dosimetric measurement setups featuring body phantoms and robotic sensor scans have ascertained correct modeling of applicator performance. Thermal measurements have been performed using a variety of methods such as infrared thermometry or invasive and non-invasive thermal catheter measurements on phantoms, volunteers, and patients. Clinical outcome has been statistically compared with simulated dose quantities and the prediction of critical hot-spots has been correlated with patient and measurement feedback.
Procedure Overview
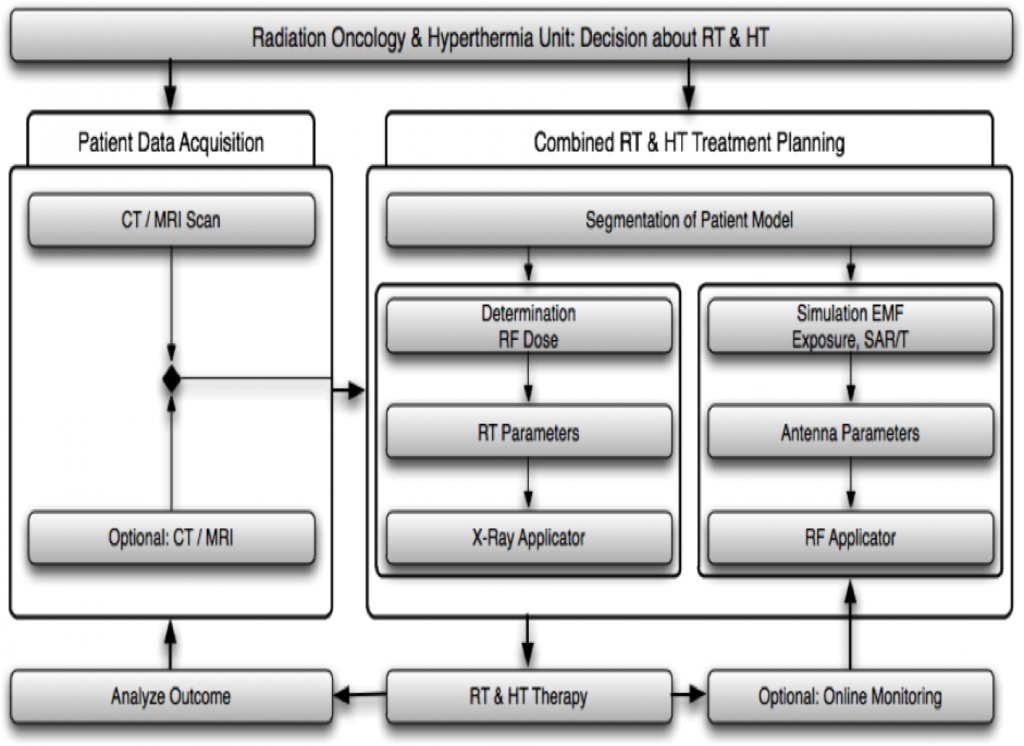
Clinical integration of hyperthermia cancer treatment and hyperthermia treatment planning
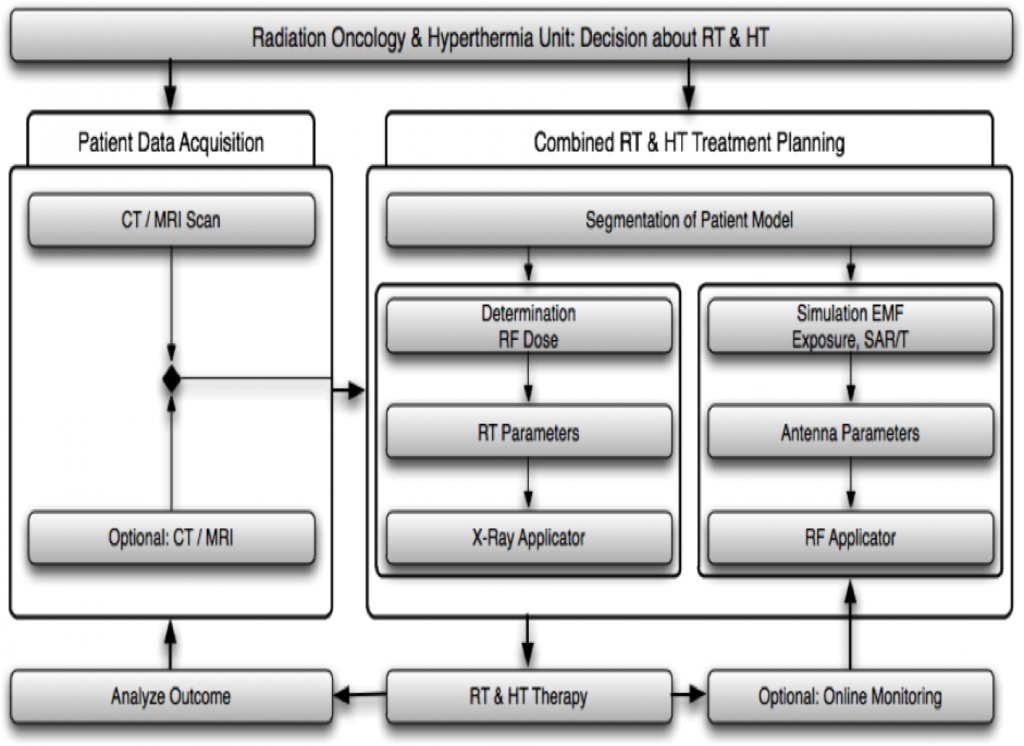
Steps in hyperthermia treatment planning and modeling
Publications
- van der Zee, Jill. “Heating the patient: a promising approach?.” Annals of oncology 13.8 (2002): 1173-1184.
- Neufeld, Esra. High resolution hyperthermia treatment planning. Hartung-Gorre Verlag, 2008.
- Neufeld, Esra. “Numerical modeling for simulation and treatment planning of thermal therapy.” Physics of Thermal Therapy: Fundamentals and Clinical Applications (2012): 119.
- Paulides, Margarethus M., et al. “Simulation techniques in hyperthermia treatment planning.” International Journal of Hyperthermia 29.4 (2013): 346-357.
- Paulides, Margarethus M., et al. “The HYPERcollar: A novel applicator for hyperthermia in the head and neck.” International Journal of Hyperthermia 23.7 (2007): 567-576.
- Paulides, Margarethus M., et al. “The clinical feasibility of deep hyperthermia treatment in the head and neck: new challenges for positioning and temperature measurement.” Physics in medicine and biology 55.9 (2010): 2465.
- Togni, Paolo, et al. “Electromagnetic redesign of the HYPERcollar applicator: toward improved deep local head-and-neck hyperthermia.” Physics in medicine and biology 58.17 (2013): 5997.
- Verhaart, René F., et al. “Temperature simulations in hyperthermia treatment planning of the head and neck region.” Strahlentherapie und Onkologie 190.12 (2014): 1117-1124.
- van Rhoon, Gerard C., et al. “CEM43° C thermal dose thresholds: a potential guide for magnetic resonance radiofrequency exposure levels?.” European radiology 23.8 (2013): 2215-2227.
- Karampatzakis, Andreas, et al. “Heating characteristics of antenna arrays used in microwave ablation: A theoretical parametric study.” Computers in biology and medicine 43.10 (2013): 1321-1327.
- de Bruijne, Maarten, et al. “Effects of waterbolus size, shape and configuration on the SAR distribution pattern of the Lucite cone applicator.” International journal of hyperthermia 22.1 (2006): 15-28.
- de Bruijne, Maarten, et al. “Quantitative validation of the 3D SAR profile of hyperthermia applicators using the gamma method.” Physics in medicine and biology 52.11 (2007): 3075.
- Paulides, Margarethus M., et al. “Laboratory prototype for experimental validation of MR-guided radiofrequency head and neck hyperthermia.” Physics in medicine and biology 59.9 (2014): 2139.
- Rijnen, Zef, et al. “Clinical integration of software tool VEDO for adaptive and quantitative application of phased array hyperthermia in the head and neck.”International Journal of Hyperthermia 29.3 (2013): 181-193.