Coping with the Challenges of Designing Medical Body Area Networks
Problem Description
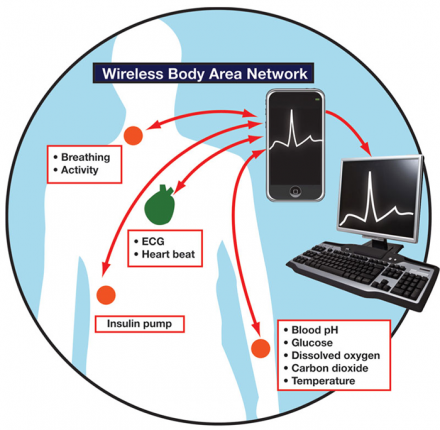
Connected nodes in a wireless body area network.
Wireless body area networks (WBAN) consist of wirelessly connected nodes of sensors or actuators, which are often enhanced with data processing. The nodes are placed in, on and around/off the human body forming a network for the continuous and unobtrusive monitoring of physiological and/or environmental signals to support medical, lifestyle and entertainment applications. Medical WBANs offer a paradigm shift from illness to wellness management, with an emphasis on early disease detection that can hopefully save some of the $4 trillion annual health care expenditure in the US. However, the design and efficient operation of WBANs bring forward several technical challenges due to the strict requirements that have to be met by the physical (PHY) layer of the applications.
Standards Addressed
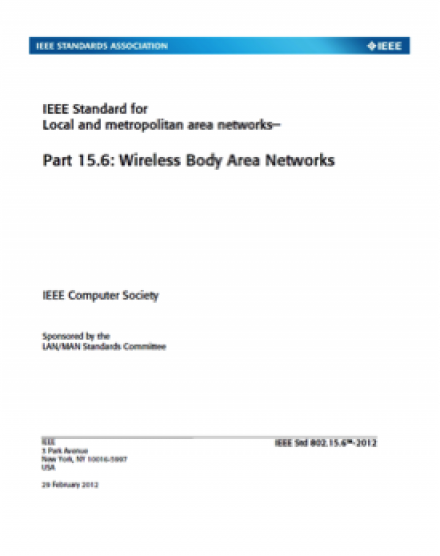
The IEEE standard 802.15.6 on Wireless Body Area Networks.
Most WBANs are built around the IEEE 802.15.6 standard on Wireless Body Area Networks, which also includes channel models based on measurements. However, these models do not account for node mobility and human interaction effects. The technical specifications for WBANs operating in Europe are described in ETSI standards EN 301 839 (402-405MHz), EN 303 203 (2483.5-2500MHz) and in technical recommendation TR 101 557 (1785-2500MHz). In all cases it is mentioned that compliance to human exposure guidelines should be checked against local regulations for basic limits on the specific absorption rate (SAR; according to IEC/TR 62630, FCC OET 65c or IEC62704-1).
Methodology
1. Technical requirements
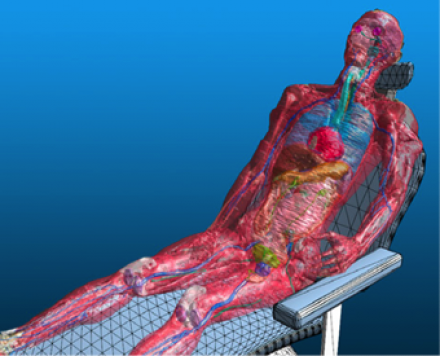
Simulation of wireless body area networks in Sim4Life.
The most critical function of a WBAN is to efficiently provide for information exchange from and to its nodes. Efficient information exchange translates to reliable, secure, fast, fault-tolerant, and interference-immune communication with low power consumption. While power consumption is outside of the scope of this document, all other aspects to achieve the desired efficiency must take into consideration many important issues closely related to the human body. Communication between implanted nodes and surface nodes (in-body and in-out communication) may experience high signal attenuation. The movements of the body parts carrying the WBAN nodes may even affect network topology by changing communication link budgets or blocking a signal. Signal propagation is highly complicated, since free space propagation is combined with diffracted, creeping and surface waves, depending on the characteristics of the source (frequency, polarization, incidence angle, body posture and shape, etc.). ZMT has developed all the necessary tools to help the designer cope with the challenges of assessing and optimizing the performance of a WBAN.
2. In-body and in-out-body RF communication
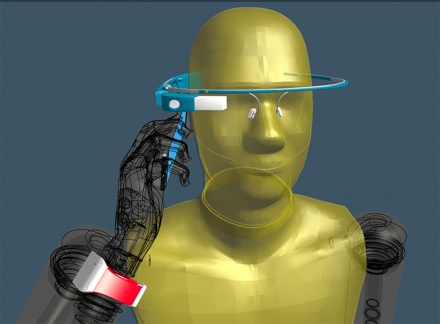
Nodes for wireless body can also comprise popular gadgets such as smart glasses and smart watches. Here they are mounted on a physical phantom for measurements, from our sister company SPEAG. Simulation allows to optimize for performance and regulatory aspects early in the design phase.
While there are simple formulas for designing free-space communications, it is impossible to characterize precisely in-body RF propagation. The latter depends on the frequency used, the position of the device, and the anatomy of the individual. Tissue inhomogeneity creates an electromagnetically complex environment, which changes with time, because of patient motion, weight variations and aging. Therefore, the use of the Virtual Population (ViP) models is the recommended solution to this challenging task. They allow for the fast placement of the BAN nodes inside any of the anatomically detailed models covering the patient population. These functionalized models naturally mimic patient movements (POSER module) and are easily morphed to extend patient population or reproduce certain pathologies. Moreover, the assignment of tissues at any frequency is straightforward with the DISFIT module. The P-EM-FDTD physics model is used to evaluate the in-body and in-out body communication performance either for narrowband or for ultra-wideband (UWB) applications.
3. Antenna design

Sim4Life stands to the challenge of simulating highly resolved CAD models with complex full-body phantoms.
Whether in or on the body, antennas used at WBAN body-nodes are usually non-resonant, due to size constraints. Therefore, the MATCH module is a valuable tool for optimizing antenna performance. Since in most cases such antennas are miniaturized, it is important to be able to model accurately their geometry (with the subgrid engine in the P-EM-FDTD model), in order to study the effects of dimensions and material properties. The impact of patient motion, node position and device orientation on the antenna characteristics (impedance, radiation pattern) is easy to assess with the posable human phantoms. Finally, with the MIMOS module the user can get a clearer picture about signal availability, since more and more hubs or base stations use antenna diversity to improve quality and reliability of the links in a WBAN.
4. Communication link budget

Electromagnetic fields generated by a body area network node in and around the human body.
Received signal strength at a node is calculated with the P-EM-FDTD model for in- and on-body nodes. In the case of large problems (hospital or home rooms) the high performance computing (HPC) framework allows for full-wave analysis of the realistic environment and the estimation of link budget between body-nodes and hubs/base-stations. If propagation data are available from other numerical techniques for sources in a complex indoor environment, the HUYGENS module offers a quick solution to establishing the link budget. The body positioning and posture of any of the ViP3.0 phantoms is easily defined in space to cover lifelike patient activity. Finally, a similar approach can be followed to investigate interference issues that arise from the coexistence of WBANs with other technologies or resolve energy detection thresholds (EDT) for clear channel assessment (CCA) protocols.
5. Regulatory compliance

CAD model of a phantom from our sister company SPEAG wearing a smart watch, simulated in Sim4Life.
Regulatory compliance of WBAN devices is complicated by the fact that the patient is free to roam across different jurisdictions in the world. However, the maximum equivalent isotropically radiated power (EIRP) to achieve desired received signal strength (RSS) levels is estimated with the highest degree of certainty with the P-EM-FDTD model and, thereafter, compared against ETSI or FCC regulations. Moreover, the peak spatial SAR distribution is generated according to the IEC62704-1 draft standard at the press of a button, to allow comparison with exposure guidelines. The MBSAR module can combine the SAR pattern from simulations at different frequency bands and compute/visualize the peak spatial SAR of the total SAR distribution. This tool is invaluable in the case the WBAN nodes are using various frequency bands.
Publications
- W. Scanlon, G. Conway, and S. Cotton, “Antennas and propagation considerations for robust wireless communications in medical body area networks,” in IET Seminar on Antennas and Propagation for Body- Centric Wireless Communications, p. 37, IET, 2007.
- G. A. Conway and W. G. Scanlon, “Antennas for over-body-surface communication at 2.45 GHz,” IEEE Trans. Antennas Propag., vol. 57, no. 4, pt. 1, pp. 844–855, Apr. 2009.
- D. Kurup, W. Joseph, G. Vermeeren, and L. Martens “In-body Path Loss Model for Homogeneous Human Tissues,” IEEE Trans. Electromagnetic Compatibility, vol.54, no.3, pp. 556-564, June 2012